გვირაბების უსაფრთხო ვენტილაცია ტრანსფორმირებადი ელემენტების გამოყენებით
რეზიუმე
ნაშრომში განხილულია, ჰორიზონტალურ და დახრილ საავტომობილო გვირაბებში სხვადასხვა მიზეზებით ინიცირებული და განვითარებული, განსხვავებული სიმძლავრის ხანძრების პირობებში ფორმირებული დამაზიანებელი ფაქტორების გვირაბში გავრცელების ხასიათი და მათი კორელაცია გვირაბის ფორმებზე, გეომეტრიაზე და დახრილობაზე, სავენტილაციო ჰაერის ნაკადის კრიტიკულ სიჩქარეზე, კვამლისა და სხვა ნამწვი პროდუქტების გვირაბში უკუდინების მანძილზე, განსაზღვრულია გვირაბში ხანძრის პირობებში სახიფათო ზონაში აღმოჩენილი ადამიანების სიცოცხლის გადარჩენისათვის აუცილებელი საევაკუაციო დროის ხანგრძლივობის მნიშვნელობა, არსებითი აქცენტია დასმული საავტომობილო გვირაბების უსაფრთხოდ ექსპლუატაციის თვალსაზრისით, გვირაბების თბოფიზიკური გაანგარიშების მნიშვნელობაზე.
შემოთავაზებულია, სახანძრო უსაფრთხოების უზრუნველყოფის მართვის სისტემების სრულყოფისა და ექსტრემალურ პირობებში აღმოჩენილი ადამიანების სიცოცხლის გადარჩენის მიზნით, მოქნილი ტრანსფორმირებადი სისტემები, რომელიც გვირაბში განვითარებული ხანძრის პირობებში, აეროდინამიკური წინაღობის გაზრდის შედეგად შეაფერხებს წვის მავნე პროდუქტების გავრცელებას ადამიანების ევაკუაციის გზაზე. აღნიშნული მოწყობილობის მოქმედების ეფექტურობა შესწავლილია რიცხვითი და ფიზიკური მოდელირების გზით სხვადასხვა დახრილობის გვირაბებისათვის განსხვავებული ხანძრის სიმძლავრისა და სცენარების პირობებისათვის.
რიცხვითი მოდელირების მეთოდით შესწავლილია, ჰაერის მიწოდების ნახევრად განივი სავენტილაციო სისტემით აღჭურვილ ჰორიზონტალურ გვირაბში, 100 მგვტ სიმძლავრის ხანძრის განვითარებისას ნახშირბადის მონოქსიდის გავრცელების უკუდინების მანძილის კორელაცია სავენტილაციო და სახანძრო არხებში ჰაერის ნაკადის სიჩქარესთან, შესწავლილია მოქნილი ტრანსფორმირებადი სისტემების ეფექტურობა შესაბამისი სასაზღვრო პირობებისათვის. შედეგები აჩვენებს, რომ ტრანსფორმირებადი სისტემების გამოყენება კვამლისა და წვის სხვა პროდუქტების გავრცელების უკუდინების მანძილის შემცირებაზე, სულ მცირე 10 % -იან ეფექტს იძლევა.
მოცემულია შეფასება და განხორციელდა შედარება, ჰაერის გრძივი განაწილების სავენტილაციო სისტემით აღჭურვილ ჰორიზონტალურ გვირაბში განვითარებული 5; 10; 30 და 50 მგვტ სიმძლავრის ხანძრის პირობებში დამაზიანებელი ფაქტორების გავრცელების ხასიათის, როცა მწყობრიდან გამოვიდა გვირაბის სავენტილაციო სისტემა და გააქტიურებულია ტრანსფორმირებადი სისტემები, შემთხვევასთან, როდესაც გვირაბი არ არის აღჭურვილი ტრანსფორმირებადი სისტემებით. რიცხვითი მოდელირების შედეგები ცხადყოფენ, რომ ტრანსფორმირებადი სისტემის მეშვეობით გვირაბის განივი კვეთის 50 %-ით გადაფარვის შემთხვევაში შესაძლებელია მნიშვნელოვანი დადებითი შედეგების მიღება წვის პროდუქტების გავრცელების შეზღუდვისა და სიცოცხლის გადარჩენის საქმეში.
სხვადასხვა სიმძლავრის 5, 10, 15, 20, 30, 50 მგვტ ხანძრებით გამოწვეული წვის პროდუქტების გავრცელება განხილულია 400 მ-მდე სიგრძის გვირაბებში, სადაც გვირაბების დახრილობა მოდელებზე 0, 1, 3, 5, 7, 9 % შეადგენს, გვირაბის განივი კვეთის ფართობია 42.5 მ2. ნაჩვენებია „ბუხრის ეფექტით“ გამოწვეული ისეთი დამაზიანებელი ფაქტორების დინამიკური ცვალებადობა, როგორებიცაა: ნახშირბადის მონოქსიდი და ტემპერატურა. მოდელირება შესრულებულია FDS პროგრამულ გარემოში სასრულ მოცულობათა მეთოდის გამოყენებით. მოდელირებული პროცესის დრო შეადგენს 180 წმ. სასრული მოცულობის მინიმალური უჯრედის ზომა არის 0.25 x 0.25 x 0.25 მ. ცეცხლის კერა განთავსებულია გვირაბის ცენტრალურ ნაწილში. მიღებული შედეგები მოცემულია გვირაბის ცენტრალური გრძივი კვეთის სიბრტყეში. სასაზღვრო პირობა მოცემული გვაქვს პორტალებს შორის სიმაღლეთა სხვაობით გამოწვეული დინამიკური წნევის ნაზრდის სახით ნორმალურ პირობებში.
შესწავლილია კრიტიკული სიჩქარის ცვალებადობა გვირაბის დახრილობის მიხედვით, აღნიშნული ცვალებადობის მახასიათებელი სიდიდე - გრადიენტ-ფაქტორი და კვამლის გავრცელების ხასიათი. აღნიშნულ სიდიდეებზე დაკვირვება ხდება გვირაბის მოდელზე ჰაერის ტემპერატურის ცვალებადობის მიხედვით. გაზომვა ხდება K ტიპის თერმოწყვილებით ღია და დახურული აღმქმელებით - გაზომილი მაქსიმალური შესაძლო ტემპერატურა 800 . თერმოწყვილები განთავსებულია გვირაბის მოდელის ჭერში გაკეთებულ ნახვრეტებში. ნახვრეტებს შორის მანძილია 5 და 10 სმ, რაც შეესაბამება ნატურაში გვირაბის სიგრძეს 5 და 10 მ. ხანძრის დამოდელება ხდება ბუნებრივი აირით. თერმოწყვილებიდან, ჰაერისა და გაზის ხარჯსაზომებიდან ანათვლების ერთდროული აღება, პირველადი ანალიზი და მონაცემების ციფრული ფორმით გადმოცემა ხდება მონაცემების შეგროვების „დატათეიქერის“ წარმოების DT85 მოწყობილობით.
წინამდებარე ნაშრომში შემოთავაზებულია მარტივი მოქნილი მოწყობილობა, რომელიც მთლიანად ან ნაწილობრივ გადაკეტავს გვირაბის განივკვეთს, დაყოფს გვირაბს შედარებით მოკლე სიგრძის მონაკვეთებად, პარალელურად მოახდენს ხანძრის კერის სრულ ან ნაწილობრივ იზოლირებას დროის იმ შუალედში, რაც საკმარისი უნდა აღმოჩნდეს სიცოცხლის გადარჩენისათვის. სპეციალური დირექტივის შესაბამისად, მითითებული დროის შუალედი არის პირველი 10 წთ ხანძრის დაწყებიდან. მაშასადამე, შემოთავაზებული მოწყობილობა გაზრდის გვირაბის აეროდინამიკურ წინაღობას, შეაფერხებს წვის პროდუქტების გავრცელებას სავენტილაციო ნაკადთან ერთად და არის სავენტილაციო ტექნოლოგიის ნაწილი. ხაზგასმით აღვნიშნავთ, რომ შემოთავაზებული მოწყობილობის პრეროგატივა არის სიცოცხლის გადარჩენაში ხელშეწყობა. თუმცა გვირაბში ანალოგიური მოწყობილობის დამონტაჟების შემთხვევაში როგორც გვირაბის მომსახურე პერსონალს, ისე მაშველებს სავენტილაციო ნაკადისა და მასთან ერთად მოძრავი წვის ტოქსიკური პროდუქტების მართვის ერთმნიშვნელოვნად უფრო მეტი საშუალება ექნებათ. აღნიშნული მოწყობილობა შესაძლებელია დამონტაჟდეს ყოველ 200-500 მ მანძილზე და რაღაცნაირად შეუთავსდეს საავარიო გასასვლელებს, რომლებიც აღნიშნულ ადგილებში უნდა მოეწყოს. ან შესაძლებელია დამონტაჟდეს საავარიო სადგურებთან ყოველ 150 მ მანძილზე ახალი გვირაბებისათვის და 250 მ მანძილზე ძველი გვირაბებისათვის, რასაც ითვალისწინებს ახალი რეგულაცია გვირაბების უსაფრთხოების შესახებ. ამგვარად, დამყოფი სისტემის განლაგება გვირაბში მოხდება სათანადო სტანდარტით განსაზღვრულ უბნებზე და გვირაბის განივი კვეთის გეომეტრიის შესაბამისად მას უნდა ექნეს ტრანსფორმირების შესაძლებლობა.
გადმოწერა
გამოყენებული ლიტერატურა
Atkinson, G., & Wu, Y. (1996). Smoke Control in Sloping Tunnels. Fire Safety Journal., pp. 335-341. Retrieved from
https://www.sciencedirect.com/science/article/abs/pii/S0379711296000616
Beard, A., & Carvel, R. (2015). The Handbook of Tunnel Fire Safety. © 2012 ICE Publishing. Doi: HTTPs://www.icevirtuallibrary.com/doi/abs/10.1680/htfs.41530
Carvel, R., Beard, A., & Jowitt, P. (2005). Fire spread between vehicles in tunnels: Effects of tunnel size, longitudinal ventilation and vehicle spacing. Fire Technology, 41 (4), pp. 271-304. Doi: HTTPs://doi.org/10.1007/s10694-005-4050-y
Chow, W., Gao, Y., Zhao, J., Dang, J., Chow, C., & Miao, L. (2015). Smoke movement in tilted tunnel fires with longitudinal ventilation. Fire Safety Journal., PP. 12-24. Retrieved from https://www.sciencedirect.com/science/article/abs/pii/S0379711215000533
Comity technique 5 Tunnels routers / Technical Committee 5 Road Tunnels. (1999). Fire and Smoke Control in Road Tunnels. PIARC Ref.: 05.05.BEN. Retrieved from https://www.piarc.org/en/order-library/3854-en-Fire%20and%20Smoke%20Control%20in%20Road%20Tunnels
Danziger, N., & Kennedy, W. (1982). Longitudinal Ventilation Analysis for the Glenwood Canyon Tunnels. Proceedings of the Fourth International Symposium Aerodynamics and Ventilation of Vehicle Tunnels. (pp. pp. 169–186). York, England.: Scientific Research Publishing.
de Groot, S., & Mazur, R. (2013). Non-equilibrium thermodynamics. Dover Publications Inc.
Economic Commission for Europe, Inland Transport Committee (2001). Report of The ad Hoc Multidisciplinary Group of Experts on Safety in Tunnels on Its Fifth Session. United Nations, Economic and Social Council. Retrieved from
https://unece.org/DAM/trans/doc/2001/sc1/TRANS-SC1-2001-12e.pdf
Economic Commission for Europe, Inland Transport Committee (2002). Report of The ad Hoc Multidisciplinary Group of Experts on Safety in Tunnels on Its Fifth Session. United Nations, Economic and Social Council. Retrieved from
https://unece.org/fileadmin/DAM/trans/doc/2002/ac7/TRANS-AC7-11e.pdf
Gannouni, S., & Maad, R. (2015). Numerical study of the effect of blockage on critical velocity and backlayering length in longitudinally ventilated tunnel fires. Tunnelling and Underground Space Technology 48, pp. 147-155.
Doi: HTTPs://doi.org/10.1016/j.tust.2015.03.003.
Heselden, A. (1976). Studies of fire and smoke behaviour relevant to tunnels. Building Research Establishment, Fire Research Station, Borehamwood. Retrieved from https://www.worldcat.org/title/studies-of-fire-and-smoke-behaviour-relevant-to-tunnels/oclc/6048884
Hinkley, P. (1970). The Flow of Hot Gases Along an Enclosed Shopping Mall a Tentative Theory. Fire Safety Science Digital Archive, Fire Research Notes 807. Retrieved from https://publications.iafss.org/publications/frn/807/-1
Hwang, C., & Edwards, J. (2004). The critical ventilation velocity in tunnel fires a computer simulation. Fire Safety Journal 40 (3), pp. 213-244. Doi: HTTPs://doi.org/10.1016/j. firesaf.2004.11.001
Ilias, N., Lanchava, O., & Nozadze, G. (2016). Numerical Modelling of First for Road Tunnels with Longitudinal Ventilacion System. International Multidisciplinary Symposium "Universitaria Simpro 2016". (pp. pp 77-80). Petrosani: Quality-Access to Success, 18 (S1). https://www.srac.ro/calitatea/en/arhiva_supplements.html#2017
Ilias, N., Lanchava, O., & Nozadze, G. (2020). Numerical simulation of air flow in short metro ventilation shafts caused by a piston effect. MATEC Web of Conferences 305, 00050
Ilias, N., Lanchava, O., Nozadze, G., & Tsanava, D. (2021). Study of propagation of harmful factors of fire in short road tunnels with different inclinations. 9th edition of the International Multidisciplinary Symposium “UNIVERSITARIA SIMPRO 2021". (p. 9). MATEC Web of Conferences 342, 03023.
Doi: https://doi.org/10.1051/matecconf/202134203023
Ingason, H., & Li, Y. (2011). Model scale tunnel fire tests with point extraction ventilation. Journal of Fire Protection Engineering, Volume 21.
Doi: HTTPs://doi.org/10.1177/1042391510394242
Jiang, X., Zhang, H., & Jing, A. (2018). Effect of blockage ratio on critical velocity in tunnel model fire tests. Tunnelling and Underground Space Technology 82, pp. 584-591. Doi: https://doi.org/ 10.1016/j.tust.2018.09.001
Khieu, H. T., Lee, Y., Kim, J., & Ryou, H. (2020). Numerical Study of the Effects of the Jet Fan Speed, Heat Release Rate and Aspect Ratio on Smoke Movement in Tunnel Fires. Energies 2020, 13(5)., pp. 1206. Doi: HTTPs://doi.org/10.3390/en13051206
Khokerashvili, Z., Tsanava., Machavariani, N. (2022). Propagation of Carbon Monoxide in Road Tunnels in Case of Fire by Considering the Critical Velocity, Backlayering and Gradient Factor. GEORGIAN SCIENTISTS, 4(5), pp. 334–336.
Doi: HTTPs://doi.org/10.52340/gs.2022.04.05.36
Ko, Y., & Hadjisophocleous, G. V. (2013). Study of smoke backlayering during suppression in tunnels. Fire safety journal., pp. 240-247. Retrieved from https://agris.fao.org/agris-search/search.do?recordID=US201900433122
Kong, J., Xu, Z., You, W., Wang, B., Liang, Y., & Chen, T. (2021). Study of smoke backlayering length with different longitudinal fire locations in inclined tunnels under natural ventilation. Tunnelling and Underground Space Technology 107, 103663. Doi: HTTPs://doi.org/ 10.1016/j.tust.2020.103663
Kuzin, V., Velichko, A., & Khokhotva, N. (2018). Edinaia metodika prognozirovania temperaturnykh uslovii v ugol’nykh shakhtakh: pp. 196.
Lanchava, O. Kunchulia, T., Khokerashvili, Z., Arudashvili, N., Tsanava, D. (2022). Determination of Non-Stationary Heat and Mass Transfer Coefficients in Tunnels. BULLETIN OF THE GEORGIAN NATIONAL ACADEMY OF SCIENCES, vol. 16, no. 2, pp. 33-39. Retrieved from http://science.org.ge/bnas/vol-16-2.html
Lanchava, O., Makharadze, L., Nozadze, G., Jangidze, M., Javakhishvili, G., Kunchulia, T., Khokerashvili, Z., Arudashvili, N., Tsanava, D., & Sebiskveradze, S. (2022). Saving lives in a road tunnel using transformable elements restricting the propagation of fire products. GEORGIAN SCIENTISTS, 4(2). https://doi.org/10.52340/gs.2022.04.02.01
Lanchava, O. (1982). Journal of Mining Science 1(6), pp. 87-92.
Lanchava, O. (1982). Heat and mass exchange in permanent mine workings. Soviet Mining 18 (6), pp. 529-532.
Lanchava, O. (1985). Journal of Mining Science 1(5), pp. 99-104.
Lanchava, O. (1986). Heat and mass exchange in newly driven mine workings. Soviet Mining Sci. (English Transl.); (United States) 21 (5), pp. 460-464.
Lanchava, O., Ilias, N., Andras, I., Moraru, R., & Neag, I. (2007). On the Ventilation of Transport Tunnels in the Presence of a Strong (Heavy) Fire. Annals of the University of Petrosani, 9, pp. 219-227.
Lanchava, O., Nozadze, G., Bochorishvili, N., Lebanidze, Z., Arudashvili, N., Jangidze, M., & Tsikarishvili, K. (2014). Criteria for evaluation of emergency firefighting in transport tunnels. Materials of International Conference “Transport Bridge Europe-Asia”. (pp. pp. 29-34.). Tbilisi: Materials of International Conference “Transport Bridge Europe-Asia”.
Lanchava, O. (2021). ANALYSIS OF CRITICAL AIR VELOCITY FOR TUNNEL FIRES CONTROLED BY VENTILATION. GEORGIAN SCIENTISTS, 2(2). https://doi.org/10.52340/gs.2021.313
Lanchava, O., Nozadze, G., & Arudashvili, N. (2015). ANALIZE OF FATAL FIRES IN TRANSPORT TUNNELS AND MEASURES OF ITS PREVENTING. Mining Journal N 2, 85-89.
Lanchava, O., Abashidze, G., & Tsverava, D. (2017). Securing fire safety for underground structures. Quality-Access to Success 18, S1, pp. 63-66.
Lanchava, O., & Ilias, N. (2017). Some issues of thermal calculation of ventilation air for the metro. Journal of Engineering Sciences and Innovation 2 (2), pp. 92-105. Retrieved from https://jesi.astr.ro/wp-content/uploads/2018/07/30.pdf
Lanchava, O., Ilias, N., Nozadze, G., Radu, S.M., Moraru, R.I. & Khokerashvili, Z. (2017). The impact of the piston effect on the technological characteristics of ventilation in the subway tunnels. Proceedings of 8th International Symposium “Occupational Health and Safety” SESAM, 2, pp. 342-352.
Lanchava, O., & Ilias, N. (2018). Complex calculation method of temperature, mass transfer potential and relative humidity for ventilation flow in subway. Technical Sciences 3 (1), pp. 69-84.
Lanchava, O., Ilias, N., Nozadze, G., & Radu, S. M. (2019). Heat and hygroscopic mass exchange modelling for safety management in tunnels of metro. Quality Access to Success 20, S1., pp. 27-33.
Lanchava, O., Ilias, N., Nozadze, G., Radu, S.M., Moraru R.I., & Khokerashvili, Z. (2019). MODELLING THE PISTON EFFECT IN SUBWAY TUNNELS USING FIRE DYNAMICS SIMULATOR. Environmental Engineering & Management Journal (EEMJ) 18 (4).
Lanchava, O., & Ilias, N. (2020). Critical velocity analysis for safety management in case of tunnel fire. MATEC Web of Conferences 305, 00023.
Lanchava, O. (2020). THE NEW CRITERION OF SIMILARITY THERMO-AERODYNAMICS OF UNDERGROUND BUILDINGS. GEORGIAN SCIENTISTS, 2(2).
Lanchava, O., & Ilias, N. (2020). Calculation of railway tunnels ventilation. Journal of Engineering Sciences and Innovation 5 (1), 69-86.
Lanchava, O. (2021). ANALYSIS OF CRITICAL AIR VELOCITY FOR TUNNEL FIRES CONTROLED BY VENTILATION: Print version was published: Mining Journal 1(42); 2019. 126-132. GEORGIAN SCIENTISTS, 3(2). https://doi.org/10.52340/gs.2021.313
Lanchava, O., Ilias, N., Radu, S.M., Nozadze, G., Tsanava, D. (2021). Analysis of the use of transformable elements in intelligent tunnel ventilation systems. 10th International Symposium on Occupational Health and Safety (SESAM 2021). Petrosani, Romania: MATEC Web of Conferences 354, 00020. https://doi.org/10.1051/matecconf/202235400020
Lanchava, O., Ilias, N., Radu, S., Jangidze, M., & Khokerasvili, Z. (2021). Fire development study on physical models of transport tunnels. 9th edition of the International Multidisciplinary Symposium “UNIVERSITARIA SIMPRO 2021”. Petroşani: MATEC Web of Conferences 342, 03020. https://doi.org/10.1051/matecconf/202134203020
Lanchava, O., & Javakhishvili, G. (2021). Impact of strong fires on a road tunnel ventilation system. Bulletin of the Georgian National Academy of Sciences 15 (4), pp. 38-45.
Lanchava, O. (2021). THE AERODYNANIIC CALCULATION OF RAILROAD TUNNELS LINE OF MARABDA-AKHALQALAQI: Print version was published: Mining Journal 2(25), 2010. 55–59. GEORGIAN SCIENTISTS, 3(2). https://doi.org/10.52340/gs.2021.309
Lanchava, O., & Javakhishvili, G. (2022). Fire effect on tunnel ventilation according to numerical modeling results. GEORGIAN SCIENTISTS, 4(5), 337–354. https://doi.org/10.52340/gs.2022.04.05.37
Lanchava, O., Ilias, N., Radu, S., Nozadze, G., & Jangidze, M. (2022). Preventing The Spread of Combustible Products in Tunnels by Implementing a Divisible System. Environmental Engineering & Management Journal (EEMJ) 21 (4), pp. 627-635.
Lanchava, O., Nozadze, G., Tsanava, D. (2022.). FDS Modelling Results for 50-100 MW Fires in Terms of Semi-Transverse Ventilation in Road Tunnels. Bulletin of the Georgian National Academy of Sciences. T.16, N4, pp. 115-122.
Lanchava, O. (2022). Life-saving problems during fire in road tunnels . GEORGIAN SCIENTISTS, 4(5), 355–358. https://doi.org/10.52340/gs.2022.04.05.38
Lanchava, O., Ilias, N., Radu, S.M., Nozadze, G., & Tsanava D. (2022). Analysis of the use of transformable elements in intelligent tunnel ventilation systems. MATEC Web of Conferences 354, 00020.
Lee, S., & Ryou, H. (2005). An experimental study of the effect of the aspect ratio on the critical velocity in longitudinal ventilation tunnel fires. Journal of Fire Sciences 23 (2), pp. 119-138. Doi: HTTPs://doi.org/10.1177/0734904105044630
Lee, S., & Ryou, H. (2006). A numerical study on smoke movement in longitudinal ventilation tunnel fires for different aspect ratio. Building and Environment 41 (6), pp. 719-725. Doi: HTTPs://doi.org/10.1016/j.buildenv.2005.03.010
Li, J., Li, Y., Cheng, C., & Chow, W. (2019). A study on the effects of the slope on the critical velocity for longitudinal ventilation in tilted tunnels. Tunnelling and Underground Space Technology, 262-267. Doi: HTTPs://doi.org/10.1016/j.tust.2019.04.01
Li, Y., & Ingason, H. (2017). Effect of cross section on critical velocity in longitudinally ventilated tunnel fires. Fire Safety Journal 91, pp. 303-311. Doi: HTTPs://doi.org/10.1016/j. firesaf.2017.03.069
Li, Y., & Ingason, H. (2018). Discussions on critical velocity and critical Froude number for smoke control in tunnels with longitudinal ventilation. Fire Safety Journal, 22-26. Retrieved from
http://www.diva-portal.org/smash/record.jsf?pid=diva2%3A1236840&dswid=-3047
Li, Y., Ingason, H., & Jiang, L. (2018). Influence of tunnel slope on smoke control. Safety and Transport Fire Research. Retrieved from
https://www.diva-portal.org/smash/get/diva2:1270845/FULLTEXT02
Li, Y., Lei, B., & Ingason, H. (2010). Study of critical velocity and backlayering length in longitudinally ventilated tunnel fires. Fire Safety Journal 45 (6), pp. 361-370. https://doi.org/ 10.1016/j.firesaf.2010.07.003
Li, Y., Lei, B., & Ingason, H. (2013). Theoretical and experimental study of critical velocity for smoke control in a tunnel cross-passage. Fire Technology 49 (2), pp. 435-449. Doi: HTTPs:// doi.org/10.1007/s10694-010-0170-0
Li, Y., Lei, B., & Ingason, H. (n.d.). Study of critical velocity and backlayering length in longitudinally ventilated tunnel fires. Fire Safety Journal 45 (6), pp. 361-370. https://doi.org/ 10.1016/j.firesaf.2010.07.003
Liang, H., Pang, L., & Gao, D. (2020). A numerical study on critical ventilation velocity in slope tunnel fire under vehicle blockage. Civil Engineering Design 2 (1–2), pp. 12-19. https:// doi.org/10.1002/cend.201900009
Liu, C., Zhong, M., Song, S., Xia, F., Tian, X., Yang, Y., & Long, Z. (2020). Experimental and numerical study on critical ventilation velocity for confining fire smoke in metro connected tunnel. Tunnelling and Underground Space Technology 97, 103296.
Doi: HTTPs://doi.org/%2010.1016/j.tust.2020.103296
Lou, B., Rifu, Q., Hu, E., Jiyun, Q., & Zhenwen, H. (2020). Influence of tunnel curvature on smoke spread in a tunnel fire. Journal of the Chinese Institute of Engineers 43 (4), pp. 386-396. Doi: HTTPs://doi. org/10.1080/02533839.2020.1719897
Meng, N., Hu, X., & Tian, M. (2020). Effect of blockage on critical ventilation velocity in longitudinally ventilated tunnel fires. Tunnelling and Underground Space Technology 106, 103580. Doi: HTTPs://doi.org/10.1016/j.tust.2020.103580
National Fire Protection Association, NFPA 502. (2014). Standard for road tunnels, bridges, and other limited access highways. National Fire Protection Association NFPA. Retrieved from https://www.nfpa.org/codes-and-standards/all-codes-and-standards/list-of-codes-and-standards/detail?code=502
National Fire Protection Association, NFPA 502. (2011). Standard for road tunnels, bridges, and other limited access highways. National Fire Protection Association, NFPA. Retrieved from https://www.nfpa.org/codes-and-standards/all-codes-and-standards/list-of-codes-and-standards/detail?code=502
Oka, Y., & Atkinson, G. (1955). Control of smoke flow in tunnel fires. . Fire Safety Journal 25 (4), pp. 305-322. https://doi.org/10.1016/0379-7112(96)00007-0
Omar Lanchava, & Shurman Oniani. (2021). HYGROSCOPIC POTENTIAL OF MASS TRANSFER IN ROCK MASS. GEORGIAN SCIENTISTS, 2(2). https://doi.org/10.52340/gs.02.20.293
Onsager, L. (1931). Reciprocal Relations in Irreversible Processes. Physical Review 37, pp. 405-426. Doi: HTTPs://doi.org/10.1103/PhysRev.37.405
Patil, P., Kumbarwadi, N., & Momoniat, E. (2018). Influence of chemically reactive species and a volumetric heat source or sink on mixed convection over an exponentially decreasing mainstream. Heat Transfer- Asian Res. 47 (1), pp. 111-125.
Doi: HTTPs://doi.org/10.1002/htj.21294
Peng, M., Shi, L., He, K., Yang, H., Cong, W., Cheng, X., & Richard, Y. (2020). Experimental study on fire plume characteristics in a subway carriage with doors. . Fire Technology, 56 (2), pp. 401–423. Doi: HTTPs://doi.org/10.1007/s10694-019-00882-0
Savalanpour, H., Farhanieh, B., & Afshin, H. (2019). Numerical simulation to investigate the induced buoyant flow characteristics caused by intensive heat in complex curvilinear geometries. Heat Transfer- Asian Res. 48 (3), pp. 835- 853.
Doi: HTTPs://doi.org/10.1002/ htj.21408
Savalanpour, H., Farhanieh, B., & Afshin, H. (2021). Proposing a general formula to calculate the critical velocities in tunnels with different cross-sectional shapes. Tunnelling and Underground Space Technology. Retrieved from
https://www.sciencedirect.com/science/article/abs/pii/S0886779820307525
Shafee, S., & Yozgatligil, A. (2018). An analysis of tunnel fire characteristics under the effects of vehicular blockage and tunnel inclination. Tunnelling and Underground Space Technology 79, pp.274-285. Doi: HTTPs://doi.org/10.1016/j.tust.2018.05.019
Tang, F., Lianjian, L., Wenkang, C., Changfa, T., & Zhi, Z. (2017). Studies on ceiling maximum thermal smoke temperature and longitudinal decay in a tunnel fire with different transverse gas burner locations. Applied Thermal Engineering, pp. 1674-1681. Retrieved from
https://www.sciencedirect.com/journal/applied-thermal-engineering/vol/110?page=2
Tang, Z., Liu, Y., Yuan, J., & Fang, Z. (n.d.). Study of the critical velocity in tunnels with longitudinal ventilation and spray systems. Fire Safety Journal 90, pp. 139-147. Doi: HTTPs://doi.org/ 10.1016/j.firesaf.2017.03.001
The European Parliament and The Council of The European Union, D. 2. (2004). on minimum safety requirements for tunnels in the Trans-European Road Network. Official Journal of the European Communities. Retrieved from https://eur-lex.europa.eu/legal-content/EN/TXT/?uri=celex%3A32004L0054
Thomas, P. (1958). The movement of buoyant fluid against a stream and the venting of underground fires. Fire Research Notes. Retrieved from
https://publications.iafss.org/publications/frn/351/-1/view/frn_351.pdf
Tian, X., Liu, C., & Zhong, M. (2021). Numerical and experimental study on the effects of a ceiling beam on the critical velocity of a tunnel fire based on virtual fire source. International Journal of Thermal Sciences 159.
Doi: HTTPs://doi.org/10.1016/j.ijthermalsci.2020.106635
Tilley, N., Rauwoens, P., & Merci, B. (2011). Verification of the accuracy of CFD simulations in small-scale tunnel and atrium fire configurations. Fire Safety Journal 46 (4)., pp. 186-193. Doi: HTTPs://doi.org/10.1016/j.firesaf.2011.01.007
Vaitkevicius, A., & Carvel, R. (2015). Investigating the Throttling Effect in Tunnel Fires. Fire Technology, Volume 58., pp 1619–1628. Doi: HTTPs://doi.org/10.1007/s10694-015-0512-z
Wang, F., & Wang, M. (2016). A computational study on effects of fire location on smoke movement in a road tunnel. Tunnelling and Underground Space Technology 51., pp. 405-413. Doi: HTTPs:// doi.org/10.1016/j.tust.2015.09.008
Wang, F., Wang, M., Carvel, R., & Wang, Y. (2017). Numerical study on fire smoke movement and control in curved road tunnels. Tunnelling and Underground Space Technology 67., PP. 1-7. Doi: HTTPs://doi.org/10.1016/j.tust.2017.04.015
Weng, M., Lu, X., Liu, F., Shi, X., & Yu, L. (2015). Prediction of back layering length and critical velocity in metro tunnel fires. Tunnelling and Underground Space Technology 47, pp. 64-72. Doi: HTTPs://doi.org/10.1016/j.tust.2014.12.010
Wu, Y., & Bakar, M. (2000). Control of smoke flow in tunnel fires using longitudinal ventilation systems – a study of the critical velocity. Fire Safety Journal. Doi: HTTPs://doi.org/10.1016/S0379-7112(00)00031-X
Zhang, X., Lin, Y., Shi, C., & Zhang, J. (2021). Experimental investigation on the smoke back-layering length in a branched tunnel fire considering different longitudinal ventilations and fire locations. Case Studies in Thermal Engineering, 28. Doi: HTTPs://doi.org/10.1016/j.csite.2021.101497
კუნჭულია თ., ხოკერაშვილი ზ., არუდაშვილი ნ., & ლანჩავა ო. . (2021). სავენტილაციო ნაკადების დინამიკისა და გვირაბების გეომეტრიის გავლენის შეფასება საევაკუაციო პერიოდზე საავტომობილო გვირაბის მოდელებზე, დამოდელებული ხანძრების შემთხვევაში: პროექტი განხორციელდა შოთა რუსთაველის ეროვნული სამეცნიერო ფონდის ფინანსური ხელშეწყობით (გრანტი AR-19-1936). ქართველი მეცნიერები, 1(1). https://doi.org/10.52340/gs.02.09.227
ლანჩავა ო., მახარაძე ლ. ., ნოზაძე გ. ., ჯანგიძე მ., ჯავახიშვილი გ., კუნჭულია თ., ხოკერაშვილი ზ., არუდაშვილი ნ., ცანავა დ., & სებისკვერაძე ს. (2022). სიცოცხლის გადარჩენა საავტომობილო გვირაბში ხანძრის პროდუქტების გავრცელების შემზღუდავი ტრანსფორმირებადი ელემენტების გამოყენებით. ქართველი მეცნიერები, 4(2), 1–29. https://doi.org/10.52340/gs.2022.04.02.01
ლანჩავა, ო., ნოზაძე, გ., არუდაშვილი, ნ., & ხოკერაშვილი, ზ. (2017). სითბური ფაქტორის მიხედვით თბილისის მეტროს სავენტილაციო ჰაერის გაანგარიშება. სამთო ჟურნალი, 1(38), გვ. 92-95.
ცანავა დ. (2023). მოდელურ გვირაბში ხანძრის განვითარების სცენარების ანალიზი სიცოცხლის გადარჩენის მიზნით რეალურ მიწისქვეშა სივრცეში . ქართველი მეცნიერები, 5(1), 289–302. https://doi.org/10.52340/gs.2023.05.01.25
ხოკერაშვილი ზ., & ცანავა დ. (2022). ხანძრის ფიზიკური მოდელირების შედეგები საავტომობილო გვირაბებისათვის. ქართველი მეცნიერები, 4(2), 30–45. https://doi.org/10.52340/gs.2022.04.02.02
ხოკერაშვილი ზ., მაჭავარიანი ნ., არუდაშვილი ნ., & ცანავა დ. (2022). ტრანსფორმირებადი ელემენტებით აღჭურვილ საავტომობილო გვირაბში ხანძრის განვითარების ანალიზი რიცხვითი მოდელირების მეთოდით . ქართველი მეცნიერები, 4(5), 359–362. https://doi.org/10.52340/gs.2022.04.05.39
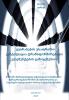
Downloads
გამოქვეყნების თარიღი
კატეგორიები
License

This work is licensed under a Creative Commons Attribution-NonCommercial-NoDerivatives 4.0 International License.