Safe ventilation of tunnels using transformable elements
Synopsis
The paper considers the nature of the damaging factors formed during the fires of different strengths, initiated and developed in horizontal and variously inclined road tunnels, and their relationship with tunnel shape, geometry, inclination, critical velocity of air flow, as well as backlayering distance of smoke and other combustion products in the tunnel. The value of the duration of evacuation time necessary to save the lives of people in the danger zone during a fire in a tunnel is calculated.
In order to improve the fire safety management systems and rescue the lives of people in extreme conditions, flexible transformable systems are proposed, which during a fire in a tunnel, at the expense of the increased aerodynamic resistance, will prevent the spread of harmful combustion products on the way of evacuation of people. The efficiency of the above device is studied by numerical and physical modelling for tunnels with different gradients for different fire strengths and scenarios.
A numerical modelling method was used to study the correlation between the backlayering distance of the carbon monoxide propagation and the air flow velocity in the ventilation and fire ducts and the efficiency of flexible transformable systems for the corresponding boundary conditions in case of a 100 MW fire in a horizontal tunnel equipped with a semi-transverse air ventilation system. The results show that the use of transformable systems gives at least 10% of the effect in reducing the backlayering distance of propagation of smoke and other combustion products.
The nature of the propagation of damaging factors in a horizontal tunnel equipped with a longitudinal air distribution ventilation system in case of 5, 10, 30 and 50 MW fires when the tunnel ventilation system fails and transformable systems are activated is assessed and compared to the tunnel scenario without transformable systems. The results of the numerical simulations show that when 50% of the tunnel cross section is closed by a transformable system, significant positive results in limiting the spread of combustion products and saving human lives can be obtained.
The propagation of combustion products from fires of different strengths of 5, 10, 15, 20, 30, 50 MW is considered in tunnels up to 400 m long, with tunnel inclinations on models of 0, 1, 3, 5, 7, 9% and tunnel cross-sectional area of 42.5 m2. The dynamic change of the damaging factors such as carbon monoxide and temperature due to the “chimney effect” is demonstrated. Modelling is performed with FDS software using a finite-volume method. The time of the simulated process is 180 s. The minimum finite volume cell size is 0.25 x 0.25 x 0.25 m. The fire seat is located in the central part of the tunnel. The obtained results are given in the plane of the central longitudinal section of the tunnel. The boundary condition is given as an increase in dynamic pressure caused by the height difference between the portals in normal conditions.
The variation of the critical velocity depending on the tunnel slope, characteristic value of this variation - the gradient coefficient, and the pattern of smoke propagation are studied. The said values are observed on the tunnel model for varying air temperatures. Measurements are made with K-type thermocouples with open and closed sensors. The maximum possible temperature measured is 800°C. The thermocouples are placed in holes made in the ceiling of the model tunnel. The distance between the holes is 5 and 10 cm, which corresponds to the natural tunnel length of 5 and 10 m. The fire is simulated by natural gas. Simultaneous readings from thermocouples, as well as air and gas flow meters, primary analysis and data digitalization are done with data taker DT85.
The present paper proposes a simple flexible device that will allow a section of the tunnel to be completely or partially closed off, divide the tunnel into relatively short sections and simultaneously completely or partially isolate the seat of fire within the time interval sufficient to save lives. According to a special directive, this time interval is the first 10 minutes from the onset of fire. So, the proposed device will increase the aerodynamic resistance of the tunnel, prevent the spread of combustion products together with the ventilation flow and is a part of the ventilation system. We would like to emphasize that the prerogative of the proposed device is to help save lives. However, if such a device is installed in the tunnel, both the tunnel staff and the lifeguards will definitely have more opportunities to control the ventilation flow and the toxic combustion products moving together with it. The above-mentioned device may be installed every 200 to 500 m and somehow aligned with the tunnel emergency exits, which must be provided at specified locations. Or, it can be installed near the emergency stations at 150 m intervals for new tunnels and 250 m intervals for old tunnels, as provided for in the new tunnel safety regulations. In this way, the partitioning system will be placed in a tunnel in sections defined by the relevant standards and it must be transformable depending on the geometry of a tunnel cross-section.
Downloads
References
Atkinson, G., & Wu, Y. (1996). Smoke Control in Sloping Tunnels. Fire Safety Journal., pp. 335-341. Retrieved from
https://www.sciencedirect.com/science/article/abs/pii/S0379711296000616
Beard, A., & Carvel, R. (2015). The Handbook of Tunnel Fire Safety. © 2012 ICE Publishing. Doi: HTTPs://www.icevirtuallibrary.com/doi/abs/10.1680/htfs.41530
Carvel, R., Beard, A., & Jowitt, P. (2005). Fire spread between vehicles in tunnels: Effects of tunnel size, longitudinal ventilation and vehicle spacing. Fire Technology, 41 (4), pp. 271-304. Doi: HTTPs://doi.org/10.1007/s10694-005-4050-y
Chow, W., Gao, Y., Zhao, J., Dang, J., Chow, C., & Miao, L. (2015). Smoke movement in tilted tunnel fires with longitudinal ventilation. Fire Safety Journal., PP. 12-24. Retrieved from https://www.sciencedirect.com/science/article/abs/pii/S0379711215000533
Comity technique 5 Tunnels routers / Technical Committee 5 Road Tunnels. (1999). Fire and Smoke Control in Road Tunnels. PIARC Ref.: 05.05.BEN. Retrieved from https://www.piarc.org/en/order-library/3854-en-Fire%20and%20Smoke%20Control%20in%20Road%20Tunnels
Danziger, N., & Kennedy, W. (1982). Longitudinal Ventilation Analysis for the Glenwood Canyon Tunnels. Proceedings of the Fourth International Symposium Aerodynamics and Ventilation of Vehicle Tunnels. (pp. pp. 169–186). York, England.: Scientific Research Publishing.
de Groot, S., & Mazur, R. (2013). Non-equilibrium thermodynamics. Dover Publications Inc.
Economic Commission for Europe, Inland Transport Committee (2001). Report of The ad Hoc Multidisciplinary Group of Experts on Safety in Tunnels on Its Fifth Session. United Nations, Economic and Social Council. Retrieved from
https://unece.org/DAM/trans/doc/2001/sc1/TRANS-SC1-2001-12e.pdf
Economic Commission for Europe, Inland Transport Committee (2002). Report of The ad Hoc Multidisciplinary Group of Experts on Safety in Tunnels on Its Fifth Session. United Nations, Economic and Social Council. Retrieved from
https://unece.org/fileadmin/DAM/trans/doc/2002/ac7/TRANS-AC7-11e.pdf
Gannouni, S., & Maad, R. (2015). Numerical study of the effect of blockage on critical velocity and backlayering length in longitudinally ventilated tunnel fires. Tunnelling and Underground Space Technology 48, pp. 147-155.
Doi: HTTPs://doi.org/10.1016/j.tust.2015.03.003.
Heselden, A. (1976). Studies of fire and smoke behaviour relevant to tunnels. Building Research Establishment, Fire Research Station, Borehamwood. Retrieved from https://www.worldcat.org/title/studies-of-fire-and-smoke-behaviour-relevant-to-tunnels/oclc/6048884
Hinkley, P. (1970). The Flow of Hot Gases Along an Enclosed Shopping Mall a Tentative Theory. Fire Safety Science Digital Archive, Fire Research Notes 807. Retrieved from https://publications.iafss.org/publications/frn/807/-1
Hwang, C., & Edwards, J. (2004). The critical ventilation velocity in tunnel fires a computer simulation. Fire Safety Journal 40 (3), pp. 213-244. Doi: HTTPs://doi.org/10.1016/j. firesaf.2004.11.001
Ilias, N., Lanchava, O., & Nozadze, G. (2016). Numerical Modelling of First for Road Tunnels with Longitudinal Ventilacion System. International Multidisciplinary Symposium "Universitaria Simpro 2016". (pp. pp 77-80). Petrosani: Quality-Access to Success, 18 (S1). https://www.srac.ro/calitatea/en/arhiva_supplements.html#2017
Ilias, N., Lanchava, O., & Nozadze, G. (2020). Numerical simulation of air flow in short metro ventilation shafts caused by a piston effect. MATEC Web of Conferences 305, 00050
Ilias, N., Lanchava, O., Nozadze, G., & Tsanava, D. (2021). Study of propagation of harmful factors of fire in short road tunnels with different inclinations. 9th edition of the International Multidisciplinary Symposium “UNIVERSITARIA SIMPRO 2021". (p. 9). MATEC Web of Conferences 342, 03023.
Doi: https://doi.org/10.1051/matecconf/202134203023
Ingason, H., & Li, Y. (2011). Model scale tunnel fire tests with point extraction ventilation. Journal of Fire Protection Engineering, Volume 21.
Doi: HTTPs://doi.org/10.1177/1042391510394242
Jiang, X., Zhang, H., & Jing, A. (2018). Effect of blockage ratio on critical velocity in tunnel model fire tests. Tunnelling and Underground Space Technology 82, pp. 584-591. Doi: https://doi.org/ 10.1016/j.tust.2018.09.001
Khieu, H. T., Lee, Y., Kim, J., & Ryou, H. (2020). Numerical Study of the Effects of the Jet Fan Speed, Heat Release Rate and Aspect Ratio on Smoke Movement in Tunnel Fires. Energies 2020, 13(5)., pp. 1206. Doi: HTTPs://doi.org/10.3390/en13051206
Khokerashvili, Z., Tsanava., Machavariani, N. (2022). Propagation of Carbon Monoxide in Road Tunnels in Case of Fire by Considering the Critical Velocity, Backlayering and Gradient Factor. GEORGIAN SCIENTISTS, 4(5), pp. 334–336.
Doi: HTTPs://doi.org/10.52340/gs.2022.04.05.36
Ko, Y., & Hadjisophocleous, G. V. (2013). Study of smoke backlayering during suppression in tunnels. Fire safety journal., pp. 240-247. Retrieved from https://agris.fao.org/agris-search/search.do?recordID=US201900433122
Kong, J., Xu, Z., You, W., Wang, B., Liang, Y., & Chen, T. (2021). Study of smoke backlayering length with different longitudinal fire locations in inclined tunnels under natural ventilation. Tunnelling and Underground Space Technology 107, 103663. Doi: HTTPs://doi.org/ 10.1016/j.tust.2020.103663
Kuzin, V., Velichko, A., & Khokhotva, N. (2018). Edinaia metodika prognozirovania temperaturnykh uslovii v ugol’nykh shakhtakh: pp. 196.
Lanchava, O. Kunchulia, T., Khokerashvili, Z., Arudashvili, N., Tsanava, D. (2022). Determination of Non-Stationary Heat and Mass Transfer Coefficients in Tunnels. BULLETIN OF THE GEORGIAN NATIONAL ACADEMY OF SCIENCES, vol. 16, no. 2, pp. 33-39. Retrieved from http://science.org.ge/bnas/vol-16-2.html
Lanchava, O., Makharadze, L., Nozadze, G., Jangidze, M., Javakhishvili, G., Kunchulia, T., Khokerashvili, Z., Arudashvili, N., Tsanava, D., & Sebiskveradze, S. (2022). Saving lives in a road tunnel using transformable elements restricting the propagation of fire products. GEORGIAN SCIENTISTS, 4(2). https://doi.org/10.52340/gs.2022.04.02.01
Lanchava, O. (1982). Journal of Mining Science 1(6), pp. 87-92.
Lanchava, O. (1982). Heat and mass exchange in permanent mine workings. Soviet Mining 18 (6), pp. 529-532.
Lanchava, O. (1985). Journal of Mining Science 1(5), pp. 99-104.
Lanchava, O. (1986). Heat and mass exchange in newly driven mine workings. Soviet Mining Sci. (English Transl.); (United States) 21 (5), pp. 460-464.
Lanchava, O., Ilias, N., Andras, I., Moraru, R., & Neag, I. (2007). On the Ventilation of Transport Tunnels in the Presence of a Strong (Heavy) Fire. Annals of the University of Petrosani, 9, pp. 219-227.
Lanchava, O., Nozadze, G., Bochorishvili, N., Lebanidze, Z., Arudashvili, N., Jangidze, M., & Tsikarishvili, K. (2014). Criteria for evaluation of emergency firefighting in transport tunnels. Materials of International Conference “Transport Bridge Europe-Asia”. (pp. pp. 29-34.). Tbilisi: Materials of International Conference “Transport Bridge Europe-Asia”.
Lanchava, O. (2021). ANALYSIS OF CRITICAL AIR VELOCITY FOR TUNNEL FIRES CONTROLED BY VENTILATION. GEORGIAN SCIENTISTS, 2(2). https://doi.org/10.52340/gs.2021.313
Lanchava, O., Nozadze, G., & Arudashvili, N. (2015). ANALIZE OF FATAL FIRES IN TRANSPORT TUNNELS AND MEASURES OF ITS PREVENTING. Mining Journal N 2, 85-89.
Lanchava, O., Abashidze, G., & Tsverava, D. (2017). Securing fire safety for underground structures. Quality-Access to Success 18, S1, pp. 63-66.
Lanchava, O., & Ilias, N. (2017). Some issues of thermal calculation of ventilation air for the metro. Journal of Engineering Sciences and Innovation 2 (2), pp. 92-105. Retrieved from https://jesi.astr.ro/wp-content/uploads/2018/07/30.pdf
Lanchava, O., Ilias, N., Nozadze, G., Radu, S.M., Moraru, R.I. & Khokerashvili, Z. (2017). The impact of the piston effect on the technological characteristics of ventilation in the subway tunnels. Proceedings of 8th International Symposium “Occupational Health and Safety” SESAM, 2, pp. 342-352.
Lanchava, O., & Ilias, N. (2018). Complex calculation method of temperature, mass transfer potential and relative humidity for ventilation flow in subway. Technical Sciences 3 (1), pp. 69-84.
Lanchava, O., Ilias, N., Nozadze, G., & Radu, S. M. (2019). Heat and hygroscopic mass exchange modelling for safety management in tunnels of metro. Quality Access to Success 20, S1., pp. 27-33.
Lanchava, O., Ilias, N., Nozadze, G., Radu, S.M., Moraru R.I., & Khokerashvili, Z. (2019). MODELLING THE PISTON EFFECT IN SUBWAY TUNNELS USING FIRE DYNAMICS SIMULATOR. Environmental Engineering & Management Journal (EEMJ) 18 (4).
Lanchava, O., & Ilias, N. (2020). Critical velocity analysis for safety management in case of tunnel fire. MATEC Web of Conferences 305, 00023.
Lanchava, O. (2020). THE NEW CRITERION OF SIMILARITY THERMO-AERODYNAMICS OF UNDERGROUND BUILDINGS. GEORGIAN SCIENTISTS, 2(2).
Lanchava, O., & Ilias, N. (2020). Calculation of railway tunnels ventilation. Journal of Engineering Sciences and Innovation 5 (1), 69-86.
Lanchava, O. (2021). ANALYSIS OF CRITICAL AIR VELOCITY FOR TUNNEL FIRES CONTROLED BY VENTILATION: Print version was published: Mining Journal 1(42); 2019. 126-132. GEORGIAN SCIENTISTS, 3(2). https://doi.org/10.52340/gs.2021.313
Lanchava, O., Ilias, N., Radu, S.M., Nozadze, G., Tsanava, D. (2021). Analysis of the use of transformable elements in intelligent tunnel ventilation systems. 10th International Symposium on Occupational Health and Safety (SESAM 2021). Petrosani, Romania: MATEC Web of Conferences 354, 00020. https://doi.org/10.1051/matecconf/202235400020
Lanchava, O., Ilias, N., Radu, S., Jangidze, M., & Khokerasvili, Z. (2021). Fire development study on physical models of transport tunnels. 9th edition of the International Multidisciplinary Symposium “UNIVERSITARIA SIMPRO 2021”. Petroşani: MATEC Web of Conferences 342, 03020. https://doi.org/10.1051/matecconf/202134203020
Lanchava, O., & Javakhishvili, G. (2021). Impact of strong fires on a road tunnel ventilation system. Bulletin of the Georgian National Academy of Sciences 15 (4), pp. 38-45.
Lanchava, O. (2021). THE AERODYNANIIC CALCULATION OF RAILROAD TUNNELS LINE OF MARABDA-AKHALQALAQI: Print version was published: Mining Journal 2(25), 2010. 55–59. GEORGIAN SCIENTISTS, 3(2). https://doi.org/10.52340/gs.2021.309
Lanchava, O., & Javakhishvili, G. (2022). Fire effect on tunnel ventilation according to numerical modeling results. GEORGIAN SCIENTISTS, 4(5), 337–354. https://doi.org/10.52340/gs.2022.04.05.37
Lanchava, O., Ilias, N., Radu, S., Nozadze, G., & Jangidze, M. (2022). Preventing The Spread of Combustible Products in Tunnels by Implementing a Divisible System. Environmental Engineering & Management Journal (EEMJ) 21 (4), pp. 627-635.
Lanchava, O., Nozadze, G., Tsanava, D. (2022.). FDS Modelling Results for 50-100 MW Fires in Terms of Semi-Transverse Ventilation in Road Tunnels. Bulletin of the Georgian National Academy of Sciences. T.16, N4, pp. 115-122.
Lanchava, O. (2022). Life-saving problems during fire in road tunnels . GEORGIAN SCIENTISTS, 4(5), 355–358. https://doi.org/10.52340/gs.2022.04.05.38
Lanchava, O., Ilias, N., Radu, S.M., Nozadze, G., & Tsanava D. (2022). Analysis of the use of transformable elements in intelligent tunnel ventilation systems. MATEC Web of Conferences 354, 00020.
Lee, S., & Ryou, H. (2005). An experimental study of the effect of the aspect ratio on the critical velocity in longitudinal ventilation tunnel fires. Journal of Fire Sciences 23 (2), pp. 119-138. Doi: HTTPs://doi.org/10.1177/0734904105044630
Lee, S., & Ryou, H. (2006). A numerical study on smoke movement in longitudinal ventilation tunnel fires for different aspect ratio. Building and Environment 41 (6), pp. 719-725. Doi: HTTPs://doi.org/10.1016/j.buildenv.2005.03.010
Li, J., Li, Y., Cheng, C., & Chow, W. (2019). A study on the effects of the slope on the critical velocity for longitudinal ventilation in tilted tunnels. Tunnelling and Underground Space Technology, 262-267. Doi: HTTPs://doi.org/10.1016/j.tust.2019.04.01
Li, Y., & Ingason, H. (2017). Effect of cross section on critical velocity in longitudinally ventilated tunnel fires. Fire Safety Journal 91, pp. 303-311. Doi: HTTPs://doi.org/10.1016/j. firesaf.2017.03.069
Li, Y., & Ingason, H. (2018). Discussions on critical velocity and critical Froude number for smoke control in tunnels with longitudinal ventilation. Fire Safety Journal, 22-26. Retrieved from
http://www.diva-portal.org/smash/record.jsf?pid=diva2%3A1236840&dswid=-3047
Li, Y., Ingason, H., & Jiang, L. (2018). Influence of tunnel slope on smoke control. Safety and Transport Fire Research. Retrieved from
https://www.diva-portal.org/smash/get/diva2:1270845/FULLTEXT02
Li, Y., Lei, B., & Ingason, H. (2010). Study of critical velocity and backlayering length in longitudinally ventilated tunnel fires. Fire Safety Journal 45 (6), pp. 361-370. https://doi.org/ 10.1016/j.firesaf.2010.07.003
Li, Y., Lei, B., & Ingason, H. (2013). Theoretical and experimental study of critical velocity for smoke control in a tunnel cross-passage. Fire Technology 49 (2), pp. 435-449. Doi: HTTPs:// doi.org/10.1007/s10694-010-0170-0
Li, Y., Lei, B., & Ingason, H. (n.d.). Study of critical velocity and backlayering length in longitudinally ventilated tunnel fires. Fire Safety Journal 45 (6), pp. 361-370. https://doi.org/ 10.1016/j.firesaf.2010.07.003
Liang, H., Pang, L., & Gao, D. (2020). A numerical study on critical ventilation velocity in slope tunnel fire under vehicle blockage. Civil Engineering Design 2 (1–2), pp. 12-19. https:// doi.org/10.1002/cend.201900009
Liu, C., Zhong, M., Song, S., Xia, F., Tian, X., Yang, Y., & Long, Z. (2020). Experimental and numerical study on critical ventilation velocity for confining fire smoke in metro connected tunnel. Tunnelling and Underground Space Technology 97, 103296.
Doi: HTTPs://doi.org/%2010.1016/j.tust.2020.103296
Lou, B., Rifu, Q., Hu, E., Jiyun, Q., & Zhenwen, H. (2020). Influence of tunnel curvature on smoke spread in a tunnel fire. Journal of the Chinese Institute of Engineers 43 (4), pp. 386-396. Doi: HTTPs://doi. org/10.1080/02533839.2020.1719897
Meng, N., Hu, X., & Tian, M. (2020). Effect of blockage on critical ventilation velocity in longitudinally ventilated tunnel fires. Tunnelling and Underground Space Technology 106, 103580. Doi: HTTPs://doi.org/10.1016/j.tust.2020.103580
National Fire Protection Association, NFPA 502. (2014). Standard for road tunnels, bridges, and other limited access highways. National Fire Protection Association NFPA. Retrieved from https://www.nfpa.org/codes-and-standards/all-codes-and-standards/list-of-codes-and-standards/detail?code=502
National Fire Protection Association, NFPA 502. (2011). Standard for road tunnels, bridges, and other limited access highways. National Fire Protection Association, NFPA. Retrieved from https://www.nfpa.org/codes-and-standards/all-codes-and-standards/list-of-codes-and-standards/detail?code=502
Oka, Y., & Atkinson, G. (1955). Control of smoke flow in tunnel fires. . Fire Safety Journal 25 (4), pp. 305-322. https://doi.org/10.1016/0379-7112(96)00007-0
Omar Lanchava, & Shurman Oniani. (2021). HYGROSCOPIC POTENTIAL OF MASS TRANSFER IN ROCK MASS. GEORGIAN SCIENTISTS, 2(2). https://doi.org/10.52340/gs.02.20.293
Onsager, L. (1931). Reciprocal Relations in Irreversible Processes. Physical Review 37, pp. 405-426. Doi: HTTPs://doi.org/10.1103/PhysRev.37.405
Patil, P., Kumbarwadi, N., & Momoniat, E. (2018). Influence of chemically reactive species and a volumetric heat source or sink on mixed convection over an exponentially decreasing mainstream. Heat Transfer- Asian Res. 47 (1), pp. 111-125.
Doi: HTTPs://doi.org/10.1002/htj.21294
Peng, M., Shi, L., He, K., Yang, H., Cong, W., Cheng, X., & Richard, Y. (2020). Experimental study on fire plume characteristics in a subway carriage with doors. . Fire Technology, 56 (2), pp. 401–423. Doi: HTTPs://doi.org/10.1007/s10694-019-00882-0
Savalanpour, H., Farhanieh, B., & Afshin, H. (2019). Numerical simulation to investigate the induced buoyant flow characteristics caused by intensive heat in complex curvilinear geometries. Heat Transfer- Asian Res. 48 (3), pp. 835- 853.
Doi: HTTPs://doi.org/10.1002/ htj.21408
Savalanpour, H., Farhanieh, B., & Afshin, H. (2021). Proposing a general formula to calculate the critical velocities in tunnels with different cross-sectional shapes. Tunnelling and Underground Space Technology. Retrieved from
https://www.sciencedirect.com/science/article/abs/pii/S0886779820307525
Shafee, S., & Yozgatligil, A. (2018). An analysis of tunnel fire characteristics under the effects of vehicular blockage and tunnel inclination. Tunnelling and Underground Space Technology 79, pp.274-285. Doi: HTTPs://doi.org/10.1016/j.tust.2018.05.019
Tang, F., Lianjian, L., Wenkang, C., Changfa, T., & Zhi, Z. (2017). Studies on ceiling maximum thermal smoke temperature and longitudinal decay in a tunnel fire with different transverse gas burner locations. Applied Thermal Engineering, pp. 1674-1681. Retrieved from
https://www.sciencedirect.com/journal/applied-thermal-engineering/vol/110?page=2
Tang, Z., Liu, Y., Yuan, J., & Fang, Z. (n.d.). Study of the critical velocity in tunnels with longitudinal ventilation and spray systems. Fire Safety Journal 90, pp. 139-147. Doi: HTTPs://doi.org/ 10.1016/j.firesaf.2017.03.001
The European Parliament and The Council of The European Union, D. 2. (2004). on minimum safety requirements for tunnels in the Trans-European Road Network. Official Journal of the European Communities. Retrieved from https://eur-lex.europa.eu/legal-content/EN/TXT/?uri=celex%3A32004L0054
Thomas, P. (1958). The movement of buoyant fluid against a stream and the venting of underground fires. Fire Research Notes. Retrieved from
https://publications.iafss.org/publications/frn/351/-1/view/frn_351.pdf
Tian, X., Liu, C., & Zhong, M. (2021). Numerical and experimental study on the effects of a ceiling beam on the critical velocity of a tunnel fire based on virtual fire source. International Journal of Thermal Sciences 159.
Doi: HTTPs://doi.org/10.1016/j.ijthermalsci.2020.106635
Tilley, N., Rauwoens, P., & Merci, B. (2011). Verification of the accuracy of CFD simulations in small-scale tunnel and atrium fire configurations. Fire Safety Journal 46 (4)., pp. 186-193. Doi: HTTPs://doi.org/10.1016/j.firesaf.2011.01.007
Vaitkevicius, A., & Carvel, R. (2015). Investigating the Throttling Effect in Tunnel Fires. Fire Technology, Volume 58., pp 1619–1628. Doi: HTTPs://doi.org/10.1007/s10694-015-0512-z
Wang, F., & Wang, M. (2016). A computational study on effects of fire location on smoke movement in a road tunnel. Tunnelling and Underground Space Technology 51., pp. 405-413. Doi: HTTPs:// doi.org/10.1016/j.tust.2015.09.008
Wang, F., Wang, M., Carvel, R., & Wang, Y. (2017). Numerical study on fire smoke movement and control in curved road tunnels. Tunnelling and Underground Space Technology 67., PP. 1-7. Doi: HTTPs://doi.org/10.1016/j.tust.2017.04.015
Weng, M., Lu, X., Liu, F., Shi, X., & Yu, L. (2015). Prediction of back layering length and critical velocity in metro tunnel fires. Tunnelling and Underground Space Technology 47, pp. 64-72. Doi: HTTPs://doi.org/10.1016/j.tust.2014.12.010
Wu, Y., & Bakar, M. (2000). Control of smoke flow in tunnel fires using longitudinal ventilation systems – a study of the critical velocity. Fire Safety Journal. Doi: HTTPs://doi.org/10.1016/S0379-7112(00)00031-X
Zhang, X., Lin, Y., Shi, C., & Zhang, J. (2021). Experimental investigation on the smoke back-layering length in a branched tunnel fire considering different longitudinal ventilations and fire locations. Case Studies in Thermal Engineering, 28. Doi: HTTPs://doi.org/10.1016/j.csite.2021.101497
კუნჭულია თ., ხოკერაშვილი ზ., არუდაშვილი ნ., & ლანჩავა ო. . (2021). სავენტილაციო ნაკადების დინამიკისა და გვირაბების გეომეტრიის გავლენის შეფასება საევაკუაციო პერიოდზე საავტომობილო გვირაბის მოდელებზე, დამოდელებული ხანძრების შემთხვევაში: პროექტი განხორციელდა შოთა რუსთაველის ეროვნული სამეცნიერო ფონდის ფინანსური ხელშეწყობით (გრანტი AR-19-1936). ქართველი მეცნიერები, 1(1). https://doi.org/10.52340/gs.02.09.227
ლანჩავა ო., მახარაძე ლ. ., ნოზაძე გ. ., ჯანგიძე მ., ჯავახიშვილი გ., კუნჭულია თ., ხოკერაშვილი ზ., არუდაშვილი ნ., ცანავა დ., & სებისკვერაძე ს. (2022). სიცოცხლის გადარჩენა საავტომობილო გვირაბში ხანძრის პროდუქტების გავრცელების შემზღუდავი ტრანსფორმირებადი ელემენტების გამოყენებით. ქართველი მეცნიერები, 4(2), 1–29. https://doi.org/10.52340/gs.2022.04.02.01
ლანჩავა, ო., ნოზაძე, გ., არუდაშვილი, ნ., & ხოკერაშვილი, ზ. (2017). სითბური ფაქტორის მიხედვით თბილისის მეტროს სავენტილაციო ჰაერის გაანგარიშება. სამთო ჟურნალი, 1(38), გვ. 92-95.
ცანავა დ. (2023). მოდელურ გვირაბში ხანძრის განვითარების სცენარების ანალიზი სიცოცხლის გადარჩენის მიზნით რეალურ მიწისქვეშა სივრცეში . ქართველი მეცნიერები, 5(1), 289–302. https://doi.org/10.52340/gs.2023.05.01.25
ხოკერაშვილი ზ., & ცანავა დ. (2022). ხანძრის ფიზიკური მოდელირების შედეგები საავტომობილო გვირაბებისათვის. ქართველი მეცნიერები, 4(2), 30–45. https://doi.org/10.52340/gs.2022.04.02.02
ხოკერაშვილი ზ., მაჭავარიანი ნ., არუდაშვილი ნ., & ცანავა დ. (2022). ტრანსფორმირებადი ელემენტებით აღჭურვილ საავტომობილო გვირაბში ხანძრის განვითარების ანალიზი რიცხვითი მოდელირების მეთოდით . ქართველი მეცნიერები, 4(5), 359–362. https://doi.org/10.52340/gs.2022.04.05.39
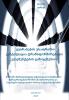
Downloads
Published
Categories
License

This work is licensed under a Creative Commons Attribution-NonCommercial-NoDerivatives 4.0 International License.