Theoretical and experimental study of subway ventilation systems to determine safe modes
Synopsis
Subway is the main transport of Tbilisi. It serves the major share of the passenger flow. Safety of mentioned transport mean is provided by all the existing systems and technical means designed for such transport. Technical means involves rolling stocks created on the bases of railway transport base and the necessary infrastructure for their movement. Subway infrastructure involves general and accessory buildings which might be located both in the over ground and underground also. In most cities of the world general buildings of subways are located under grounds and in this point of view Tbilisi subway is not an exception. For proper performance of the said transport including all related systems, a reliable performance of ventilating system is of vital importance. The purpose of it is a provision and maintenance of normal microclimate in subways in everyday basis, herewith polluted air to be returned in atmosphere and in emergency situations the system shall answer all arisen challenges. In subways, with their infrastructures located over grounds, reasons of emergency situations are mainly technogeneous and there is a quite a big risk of getting a fire emergency situation. In such situations choosing of right working regime of ventilation system has a major importance, because saving of people’s lives depends on it. Also, prevention of toxic gases and pathogenic microbes in the events of aerosol terrorist acts are very important. Mentioned danger became even actual after Tokyo (Japan) subway accident, when the group of terrorists managed to spread a poisonous gas Sarin in subway space. As a result, several people died and thousands had been poisoned. the mentioned accident the question came up quite thoroughly: Is penetration of toxic gases and pathogenic microbes in subway possible by relevant attempts? There is no qualified answer to this question for today, because there have been conducted quite a few studies and works on the issue. The abovementioned work is one of the first attempts for regulation of the issue, in specific: based on analyses of underground working systems, we established the weakest circle from where it is theoretical possibility for unplanned air to penetrate into subway. The said weakest circle is ventilation system, because it is the only direction by which underground space of subway is connected to over ground. Specifically, the most insecure points are air shafts which return polluted air from undergrounds to atmosphere, especially in cases if mentioned air shafts are not equipped with exhaust ventilation equipment. It should be noted, that none of Tbilisi (Georgia) subways are equipped with air shafts equipped with exhaust ventilation systems for polluted air. Modern computer technologies give possibility to describe created air flows resulted from piston effect of moving wagons in tunnel with high accuracy. Consequently, we made a computer modelling of the situation in Pyro Sim 2016 program. The technical characteristics of piston effect of moving wagons in the tunnel, also, speed character of transmitted and rushing air flow in gaps, in front and back sides of wagons had been revealed. There are provided specified tables and charts of their variations, by means of which and according to the geography of cross over tunnels ventilation characteristics can be established. It may be noted, that established parameters according to the abovementioned charts are in a good coincidence with figures received by theoretical counting. A new regularity has been established: a non-stationary character of air flows arisen from piston effect during the wagon movement in channel caused by length of cross over tunnel. According to the given geometry and relevant ventilation characteristics we have determined numerical indicators of excessive pressure and rarefication in front and behind of wagons correspondingly. Based on determined indicators tab-les and charts have been created. Analyses of modelling results showed the impact of air gap between perimeters of wagon and tunnel on created numismatic indicators of excessive pressure and rarefication in front and behind of wagon. Based on the abovementioned we corrected the chart describing rarefication dynamic created behind wagon for the given speed of wagon. It should be noted, that the said knot of ventilation system is really problematic. Behind wagon there is created enough rarefication which may result in getting of unplanned air to the subway area. Thus, a technological gap in ventilation system performance has been revealed, which is not a subject of insufficient quantity of ventilation air or improper quality of air, the problem is that existing ventilation systems can not perceive toxic gases or pathogenic microbes and prevent from possibilities of their penetrating and spreading in underground space. The abovementioned problematic creates a serious threat to security of metropolitan. In order to overcome the problematic, in the present work there is formulated requirements to ventilation systems for new threats and possibilities of using appropriate technical means for proper and secure performance of the problematic knot.
Downloads
References
Tsodikov. V. (1975) Ventilacia i Teplosnabjenie Metropolitenov. Moskva. “Nedra”, p. 566 (in Russian).
Yang Z., Su X., Ma F., Yu L., Wang H. (2015) An innovative environmental control system of subway. Journal of Wind Engineering and Industrial Aerodynamics 147, 120–131.
Moreno T., Reche C., Minguillón M.C., Capdevila M., de Miguel E., Querol X. (2017) The effect of ventilation protocols on airborne particulate matter in subway systems. Science of the Total Environment, 1317–1323.
Moreno T., Pérez N., Reche C., Martins V., de Miguel E., Capdevila M., Centelles S., Minguillón M.C., Amato F., Alastuey A., Querol X., Gibbons W. (2014) Subway platform air quality: Assessing the influences of tunnel ventilation, train piston effect and station design. Atmospheric Environment 92, 461 – 468
Mendes L., Gini M.I., Biskops G., Colbeck I., Eleftheriadis K. (2018) Airborne ultrafine particles in a naturally ventilated metro station: Dominant sources and mixing state determined by particle size distribution and volatility measurements. Environmental Pollution 239, 82 – 94.
Moreno T., Martins V., Querol X., Jones T., Béru Bé K., Minguillón M. C., Amato F., Capdevila M., de Miguel E., Centelles S., Gibbons W. (2015) A new look at inhalable metalliferous airborne particles on rail subway platforms. Science of the Total Environment 505, 367–375.
Wang J., Zhao L., Zhu D., Gao H. O., Xie Y., Li H., Xu X., Wang H. (2016) Characteristics of particulate matter (PM) concentrations influenced by piston wind and train door opening in the Shanghai subway system. Transportation Research. Part D 47, 77–88.
Xu B. and Hao J. (2017) Air quality inside subway metro indoor environment world-wide: A review. Environment International 107, 33–46.
Kim K.H., Ho D. X., Jeon J.S, Kim J.C. (2012) A noticeable shift in particulate matter levels after platform screen door installation in a Korean subway station. Atmospheric Environment 49, 219– 223.
Di Perna C., Carbonari A., Ansuini R., Casals M. (2014). Empirical approach for real-time estimation of air flow rates in a subway station. Tunnelling and Underground Space Technology 42, 25–39.
Yang L., Zhang Y., Xia J. (2018) Case study of train-induced airflow inside underground subway stations with simplified field test methods. Sustainable Cities and Society 37, 275–287.
Zhang Y. and Li X. (2018) A study of fresh air control in subway stations. Journal of Wind Engineering and Industrial Aerodynamics 175, 384–390.
Lia H., Tanga B., Cheb L., Liub J., Sua X. (2017) Conditioning System of Equipment Stations in Metro Systems. Procedia Engineering 205, 3519–3524.
Guo-Qing L. and Shi-Jun Y. (2011) A New System to Reduce Air Pollution in Metro Platform. Procedia Environmental Sciences 11, 1454 – 1458.
Wanga Y., Xiaofeng L. (2018), STESS: Subway thermal environment simulation software. Sustainable Cities and Society 38, 98–108.
ლანჩავა ო., ნოზაძე გ., ხოკერაშვილი ზ., არუდაშვილი ნ. (2017) სითბური ფაქტორის მიხედვით თბილისის მეტროს სავენტილაციო ჰაერის გაანგარიშება. სამთო ჟურნალი 1(38), 92-95.
Lanchava O, Ilias N. (2017) Some issues of thermal calculation of ventilation air for the metro. Journal of Engineering Sciences and Innovation 2(2), AGIR Publishing House Bucharest, Romania, 92-105.
Lanchava O., Ilias N. (2020) Calculation of railway tunnels ventilation. Journal of Engineering Sciences and Innovation 5(1), AGIR Publishing House Bucharest, Romania, 69-86.
Oniani Sh., Lanchava O. (1979) Metodika Prognozirovania Temperaturnogo Rejima Shaxt V Oslajnennix Usloviax. V Kn.: EdinnaIa Metodika Prognozirivania Uslovii V Ugolnix Shaxtax. Makeevka-Donbas. 75-92 (in Russian).
ლანჩავა ო., ხოკერაშვილი ზ., არუდაშვილი ნ. (2018) ერთობლივი სითბოსა და ჰიგროსკოპული მასის არასტაციონარული გადაცემა მეტროს სავენტილაციო ჭავლსა და გარშემომცველ სამთო მასივს შორის. სამთო ჟურნალი 1(40), 34-43.
Lanchava O., Kunchulia T., Khokerashvili Z., Arudashvili N., Tsanava D. (2022)
Determination of Non-Stationary Heat and Mass Transfer Coefficients in Tunnels. Bulletin of the Georgian National Academy of Sciences 16(2), 32-41.
Dzidziguri A., Oniani Sh., Duganov G., I dr. (1966). Teplofizichesskie svoistva gornix porod I metodi ix opredelenia. Tbilisi, Mecniereba, p. 228 (in Russian).
Lanchava O. (1998) Hygroscopic heat and mass transfer in underground structures, Tbilisi, GTU, p. 272.
Onsager L. (1931). Reciprocal Relations in Irreversible Processes. Phys. Rev. 37, 405-426.
Lanchava O., Ilias N. (2018) Complex calculation method of temperature, mass transfer potential and relative humidity for ventilation flow in subway. Journal of Engineering Sciences and Innovation 3(1), AGIR Publishing House, Bucharest, Romania, 69-84.
Lanchava O., Ilias N., Nozadze G., Radu SM. (2018) Heat and hygroscopic mass exchange modelling for safety management in tunnels of metro. “8th International Multidisciplinary Scientific Symposium” Universitaria SIMPRO. Petrosani, Romania, 27-33.
Lanchava O. (1985) Heat and mass exchange in newly driven mine workings. Sov. Min. Sci. (Engl. Transl.); (United States) 21, 460-464.
Lanchava O., Abashidze G., Tsverava D. (2017) Securing fire safety for underground structures. Supplement of Quality Access to Success; Bucharest 18(S1).
Lanchava O., Javakhishvili G. (2021) Impact of strong fires on a road tunnel ventilation system. Bulletin of the Georgian National Academy of Sciences 15(4), 38-45.
Lanchava O., Ilias N. (2020) Critical velocity analysis for safety management in case of tunnel fire. MATEC Web of Conferences 305, 00023. SESAM 2019.
კუნჭულია თ., ხოკერაშვილი ზ., არუდაშვილი ნ., ლანჩავა ზ. (2020) სავენტილაციო ნაკადების დინამიკისა და გვირაბების გეომეტრიის გავლენის შეფასება საევაკუაციო პერიოდზე საავტომობილო გვირაბის მოდელებზე, დამოდელებული ხანძრების შემთხვევაში. ჟურნალი "ქართველი მეცნიერები", 5(4), 363.
ხოკერაშვილი ზ., კუნჭულია თ., არუდაშვილი ნ., ლანჩავა ზ. (2020). სავენტილაციო ნაკადების დინამიკისა და გვირაბების გეომეტრიის გავლენის შეფასება საევაკუაციო პერიოდზე საავტომობილო გვირაბის მოდელებზე, დამოდელებული ხანძრების შემთხვევაში; მე-6 საერთაშორისო სამეცნიერო-პრაქტიკული კონფერენცია „სამთო საქმისა და გეოლოგიის განვითარება ეკონომიკის აღორძინების წინაპირობაა“ საქართველოს ტექნიკური უნივერსიტეტი. სამთო-გეოლოგიური ფაკულტეტი.
Lanchava O., Ilias N., Radu S.M., Makharadze L., Runchulia T., Arudashvili N., Khokerashvili Z. (2020) Analysis of the parameters of the fire modeled in a road tunnel. Georgian Scientists (4science.ge), 1(1).
Lanchava O., Ilias N., Radu S.M., Jangidze M., Khokerashvili Z. (2021) Fire Development Study on Physical Models of Transport Tunnels. MATEC Web Conf. 9th edition of the International Multidisciplinary Symposium “UNIVERSITARIA SIMPRO 2021”, Petrosani, Romania, 342.
ხოკერაშვილი ზ., ცანავა დ. (2022) ხანძრის ფიზიკური მოდელირების შედეგები საავტომობილო გვირაბებისათვის. ჟურნალი "ქართველი მეცნიერები" 4(2), 30-45.
Lanchava O., Makharadze L., Nozadze G., Jangidze M., Javakhishvili G., Kunchulia T., Khokerashvili Z., Arudashvili N., Tsanava D., Sebiskveradze S. (2022) Saving lives in a road tunnel using transformable elements restricting the propagation of fire products. Georgian Scientists 4(2), 1-29. https://doi.org/10.52340/gs.2022.04.02.01
Khokerashvili Z., Tsanava D. (2022) Propagation of Carbon Monoxide in Road Tunnels in Case of Fire by Considering the Critical Velocity, Backlayering and Gradient Factor. The International Scientific and Technical Conference "Problems of Engineering Sciences" Yerevan, Republic of Armenia, 31-32.
ხოკერაშვილი ზ., მაჭავარიანი ნ., არუდაშვილი ნ., ცანავა დ. (2022) ტრანსფორმირებადი ელემენტებით აღჭურვილ საავტომობილო გვირაბებში ხანძრის განვითარების ანალიზი რიცხვითი მოდელირების მეთოდით . ჟურნალი „ქართველი მეცნიერები“ 4(5), 359-362.
Lanchava O., Ilias N., Nozadze G.,Radu S., Moraru R., Khokerashvili Z., Arudashvili N. (2017) The Impact of the Piston Effect on the Technological Characteristics of Ventilation in the Subway Tunnels. Proceedings of 8th International Symposium “Occupational Health and Safety” SESAM 2017, Bucharest, Romania, 342-352.
ლანჩავა ო., ნოზაძე გ., ხოკერაშვილი ზ., არუდაშვილი ნ. (2017) დგუშის ეფექტით გამოწვეული ჰაერის ნაკადების შეფასებისათვის მეტროს გვირაბებში. სამთო ჟურნალი 2(39), 37-44.
ხოკერაშვილი ზ. (2017) მეტროს გვირაბაბში დგუშის ეფექტის მოდელირებისა და გაანგარიშების ზოგიერთი საკითხები. კონფერენცია სამთო ინსტიტუტში.
Lanchava O., Nozadze G., Khokerashvili Z. (2017) Numerical Modeling of Ventilation Flow Distribution in Subway Tunnels with Considering of the Piston Effect. Proceedings of 9th International Conference “Contemporary problems of architecture and construction”, Batumi, Georgia, 390-392.
Lanchava O., Ilias N., Radu S., Nozadze G., Javakhishvili G. (2024) Study of the throttling effect in tunnel fires. MATEC Web of Conferences 389, 00056.
Arbuzov G.V. (1950) Ventiliatsia tonnelei i metropolitenov. Transjeldorizdat, p. 88 (in Russian).
Lanchava O., Ilias N., Nozadze G., Radu S., Moraru R., Khokerashvili Z., Arudashvili N. (2019) FSD Modelling of the Piston Effect in Subway Tunnels. Environmental Engineering and Management Journal, 18(4), 317- 325.
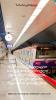
Downloads
Published
Categories
License

This work is licensed under a Creative Commons Attribution-NonCommercial-NoDerivatives 4.0 International License.